True Environmental Impact of Green Hydrogen: Emission Intensity and water usage & rewarding the lowest impactor
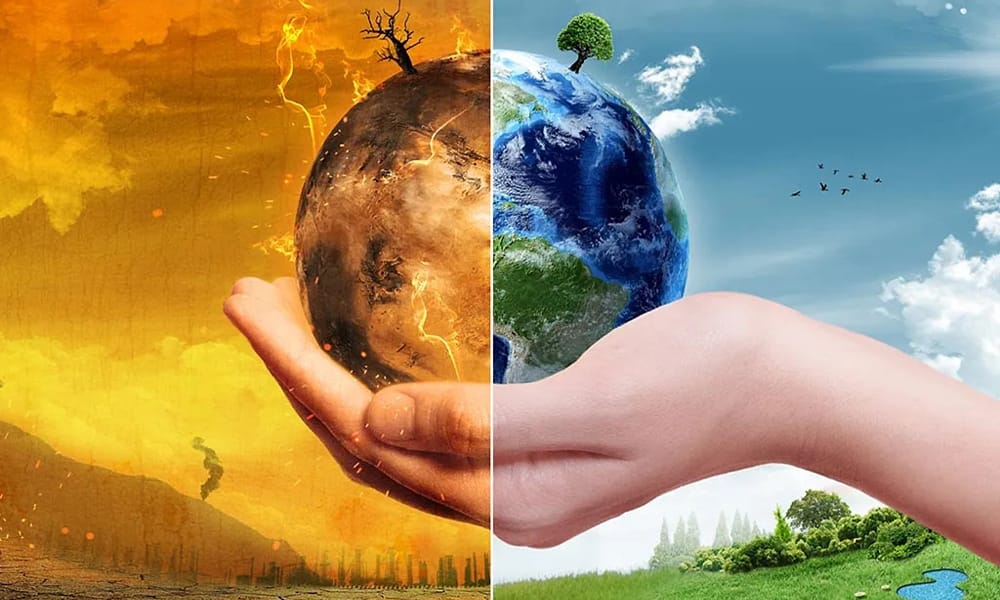
Green hydrogen has garnered attention as a critical component in the global transition toward decarbonization, offering the potential to drastically reduce greenhouse gas (GHG) emissions across industries. While its environmental benefits, particularly in terms of cutting GHG emissions, are undeniable, a comprehensive assessment of green hydrogen's environmental impact requires examining factors beyond emissions alone. Two key areas of concern are emissions intensity throughout the hydrogen production lifecycle and water usage, both of which must be addressed to ensure that green hydrogen contributes to a truly sustainable energy transition.
This write-up explores these aspects, focusing on the emissions intensity across the hydrogen production cycle, water demand, and the role of regulations and incentives in shaping sustainable hydrogen production practices.
1. Emissions Intensity Across the Hydrogen Production Cycle
Although green hydrogen production, via electrolysis powered by renewable energy, is often considered carbon-neutral, its overall environmental impact extends beyond direct GHG emissions. To evaluate the true environmental footprint of green hydrogen, it is essential to consider the entire hydrogen production lifecycle, using the widely recognized Scope 1, 2, and 3 emissions framework.
1.1 Scope 1 and 2 Emissions: Direct and Purchased Electricity Emissions
The primary factor influencing the emissions intensity of green hydrogen is the source of electricity used to power the electrolyzers that split water into hydrogen and oxygen. Electrolysis powered by renewable energy sources such as wind, solar, and hydroelectricity results in near-zero Scope 1 and Scope 2 emissions, as these energy sources do not directly produce GHG emissions during operation.
However, the sources emphasize that the grid's electricity mix is crucial. For example, electrolyzers connected to grids where a significant portion of electricity comes from fossil fuels will inherently have a higher emissions intensity compared to those powered solely by renewables. The sustainability of grid-connected green hydrogen production depends heavily on the overall decarbonization of the grid. In this case, transitioning to a greener grid—one where renewable energy becomes the dominant source—is essential for achieving low-emission hydrogen production.
To minimize emissions, many green hydrogen projects aim to connect electrolyzers directly to dedicated renewable energy sources, such as solar farms or wind turbines. This "off-grid" approach eliminates reliance on the fluctuating carbon intensity of the grid, ensuring that the hydrogen produced is as clean as possible.
1.2 Upstream Scope 3 Emissions: Material Acquisition
The Scope 3 emissions associated with green hydrogen production extend beyond the immediate production process, particularly in the upstream supply chain. Manufacturing the electrolyzers, solar panels, wind turbines, and other essential infrastructure components generates emissions, which are often overlooked in assessments of green hydrogen's environmental impact.
The production of materials such as steel, concrete, and rare-earth elements required for renewable energy infrastructure is energy-intensive and frequently involves significant GHG emissions. For instance, the production of solar panels and wind turbines, though essential for powering green hydrogen production, still relies on industrial processes that emit CO₂, especially if those processes are powered by fossil fuels.
As green hydrogen projects scale up, addressing the carbon footprint of these infrastructure components will be crucial. Innovations in material science and manufacturing processes—such as the use of low-carbon or carbon-neutral construction materials—can help reduce the upstream emissions associated with green hydrogen infrastructure.
1.3 Downstream Scope 3 Emissions: Transportation and Distribution
Transporting and distributing hydrogen, whether in its gaseous or liquefied form, also contributes to the overall emissions profile of green hydrogen. Hydrogen transportation is energy-intensive, especially for long distances, and depends on the infrastructure available. Transporting hydrogen via pipelines, trucks, or ships, for example, may require energy inputs that are not always renewable, adding to the downstream Scope 3 emissions.
To reduce the emissions associated with hydrogen transportation, the sources emphasize the need for further innovation in hydrogen logistics. This could include developing more efficient transportation technologies, integrating renewable energy into hydrogen distribution networks, or co-locating production and consumption facilities to minimize transportation needs.
2. Regulations and Incentives for Low-Emission Hydrogen
Given the challenges of reducing emissions across the hydrogen lifecycle, regulations and incentives are playing an increasingly important role in shaping the development of low-emission hydrogen technologies. Several major initiatives around the world are focused on creating frameworks that encourage the production and use of green hydrogen, while discouraging high-emission alternatives.
2.1 EU Regulations: Defining Renewable Hydrogen
In the European Union (EU), regulatory efforts have centered on defining "renewable hydrogen" based on specific emissions reduction thresholds compared to fossil fuel benchmarks. These regulations apply to both domestic hydrogen producers and international exporters to the EU, significantly impacting the design and execution of green hydrogen projects globally.
The EU's emphasis on lifecycle emissions assessments has prompted hydrogen producers to carefully consider the carbon intensity of their supply chains, including both direct emissions from production and indirect emissions from electricity generation and material acquisition. For projects aiming to sell hydrogen in the EU, meeting these stringent emissions reduction criteria is a prerequisite for market access.
2.2 European Hydrogen Bank (EHB)
The European Hydrogen Bank (EHB) is another key initiative aimed at accelerating the adoption of green hydrogen technologies by providing financial support to bridge the cost gap between renewable and fossil fuel-based hydrogen. The EHB's financial incentives are designed to offset the higher upfront costs of green hydrogen production, encouraging early adopters to invest in low-emission technologies despite their initial cost disadvantages.
By reducing the financial barriers to entry for green hydrogen projects, the EHB is helping to create a competitive market for renewable hydrogen. Over time, this is expected to drive innovation and scale, further reducing the costs of production and making green hydrogen more economically viable on a global scale.
2.3 US Inflation Reduction Act (IRA)
In the United States, the Inflation Reduction Act (IRA) provides financial benefits for hydrogen production based on the carbon intensity of the hydrogen produced. By linking financial incentives directly to emissions performance, the IRA is designed to shift investment towards cleaner hydrogen production technologies.
The sources highlight that the IRA's emphasis on carbon intensity is likely to have global implications, as international hydrogen producers may need to align their production practices with these standards in order to access the US market. By setting a high bar for emissions performance, the IRA is expected to drive further investment in low-emission hydrogen technologies worldwide.
3. Water Demand and Environmental Impacts
In addition to its emissions profile, the environmental impact of green hydrogen production is closely tied to its water footprint. Water is a critical input for electrolysis, the process used to produce green hydrogen, and large-scale hydrogen production requires significant water resources. This can be particularly challenging in water-stressed regions, where competition for water resources between industries, agriculture, and local communities is already intense.
3.1 Water Footprint of Green Hydrogen
Green hydrogen production has a smaller water footprint compared to other types of hydrogen, such as blue or grey hydrogen, which rely on fossil fuel-based processes like steam methane reforming (SMR). Nevertheless, the electrolysis process used for green hydrogen still consumes a substantial amount of water, especially at scale. For instance, it is estimated that producing 1 kilogram of hydrogen via electrolysis requires about 9 liters of water.
In arid or semi-arid regions, this level of water consumption could exacerbate existing water shortages, leading to potential conflicts over water use between hydrogen producers and other sectors. Careful water management is therefore essential to ensure that large-scale green hydrogen production does not negatively impact local water availability.
3.2 Desalination as a Water Source
In water-scarce regions, desalination has been suggested as a potential solution to meet the water needs of green hydrogen production. By converting seawater into fresh water, desalination plants could supply the water needed for electrolysis without drawing on already-limited freshwater resources.
However, desalination itself is an energy-intensive process, and integrating it into green hydrogen production adds to the overall energy consumption and cost. To mitigate this, the sources propose combining desalination plants with renewable energy sources, such as solar or wind, to reduce the environmental and economic impacts. This approach could also present opportunities for enhancing water security in regions where both water and energy are in short supply.
3.3 Integrating Water Perspectives into Hydrogen Strategies
The sources stress the importance of integrating water considerations into hydrogen strategies to ensure the sustainable use of water resources. This includes assessing the local water availability, minimizing water usage where possible, and exploring alternative water sources such as treated wastewater or naturally occurring brackish water. By diversifying water inputs and reducing reliance on freshwater, hydrogen producers can minimize potential conflicts over water use and ensure that green hydrogen production contributes to sustainable development.
Conclusion
While the push for green hydrogen is primarily driven by its potential to decarbonize industries and reduce GHG emissions, a comprehensive assessment of its environmental impact must consider the broader lifecycle emissions and water usage involved in its production and distribution. Scope 1, 2, and 3 emissions, along with water demand, are critical factors that determine the true sustainability of green hydrogen.
Addressing these environmental challenges will require a combination of technological innovation, regulatory frameworks, and incentive schemes. By focusing on both emissions intensity and water management, green hydrogen can play a pivotal role in the global energy transition while minimizing trade-offs and maximizing positive environmental impacts.