Transforming Carbon Emissions into Valuable Resources: Harnessing Green Hydrogen for Methane and Methanol Production
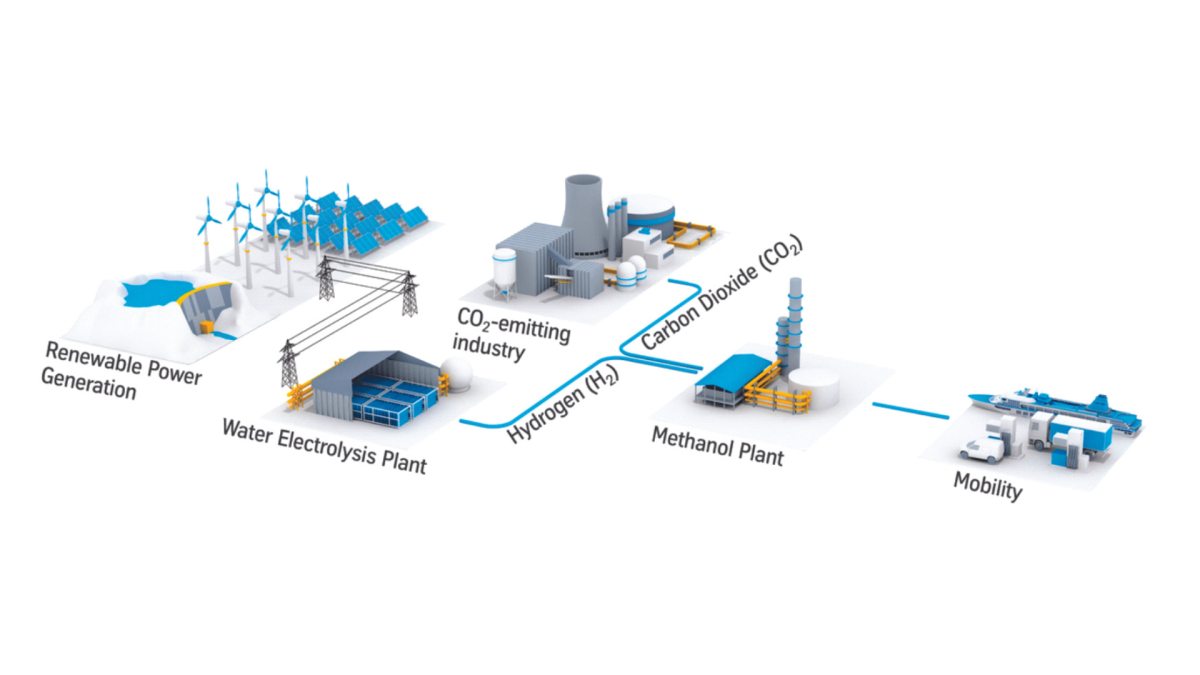
Introduction
As the global push for decarbonization intensifies, converting captured carbon into valuable resources has gained traction as an effective strategy for emissions reduction. The integration of green hydrogen in transforming carbon dioxide (CO₂) into synthetic fuels like methane and methanol is a promising development. This process involves the use of captured CO₂ from industrial sources, renewable hydrogen, and innovative conversion techniques to create eco-friendly synthetic fuels, potentially reshaping carbon management and resource utilization.
This article explores the comprehensive process of harnessing green hydrogen for methane and methanol production, reviewing each step in CO₂ capture, transportation, and conversion into synthetic fuels.
Step 1: CO₂ Point Sources – Identifying Emission Origins
The initial step in the process is pinpointing CO₂ emission sources. Typically, industrial facilities like power plants, steel mills, and cement factories emit significant quantities of CO₂ through fossil fuel combustion. These emissions contain a mix of gases, mainly nitrogen (N₂), oxygen (O₂), water vapor (H₂O), and CO₂. Identifying these CO₂ point sources is vital, as it enables the capture of emissions directly at their origin, setting the stage for efficient conversion processes.
Step 2: CO₂ Capture Units – Capturing the Carbon
CO₂ capture units are designed to extract CO₂ from the industrial flue gas. Several technologies, such as chemical absorption, membrane separation, and cryogenic distillation, have been developed to capture CO₂ efficiently. Chemical absorption remains the most widely used technique due to its maturity and effectiveness. After extraction, the CO₂-lean flue gas, which is predominantly free of CO₂, is safely released into the atmosphere, thereby reducing overall emissions.
Step 3: CO₂ Compression and Transportation
Once CO₂ has been captured, it must undergo compression and transportation, especially if conversion does not occur at the emission source.
Co-located vs. Networked Conversion
CO₂ capture and conversion can be achieved through two approaches:
- Direct Conversion at Co-located Facilities: In cases where the CO₂ conversion facility is co-located with the emission source, CO₂ transport requirements are minimized, though compression may still be necessary.
- CO₂ Transport Networks: An alternative approach involves constructing networks to link multiple CO₂ sources to centralized conversion facilities.
Compression and Pipeline Transportation
Transporting CO₂ efficiently over long distances typically requires compression to a dense phase liquid at around 15.3 MPa and 30°C. Multi-stage centrifugal compressors with intercoolers and dehydration units ensure that CO₂ remains in a manageable, non-corrosive form, essential for pipeline transportation. For co-located conversions, compression is still needed, though at lower pressures of around 15 bar.
Step 4: CO₂ Conversion to Synthetic Fuels – Methane and Methanol Production
The heart of this process lies in transforming CO₂ into valuable synthetic fuels—methanol and methane—using green hydrogen. This transformation takes advantage of CO₂ hydrogenation reactions, where CO₂ is combined with renewable hydrogen.
Methanol Production via CO₂ Hydrogenation
Traditionally, methanol production relies on syngas, a blend of carbon monoxide (CO) and hydrogen (H₂) derived from natural gas or coal. The conventional production process requires high pressures, temperatures, and specific catalysts to facilitate the reaction. The methanol synthesis from CO₂ and H₂, known as CO₂ hydrogenation, involves the following reaction:
CO₂+3H₂→CH₃OH+H₂O\text{CO₂} + 3\text{H₂} \rightarrow \text{CH₃OH} + \text{H₂O}CO₂+3H₂→CH₃OH+H₂O
The resulting methanol serves as a vital feedstock in various industries, producing formaldehyde, acetic acid, and olefins. Moreover, methanol's application as a cleaner-burning fuel can significantly reduce CO₂ emissions in transportation.
Methane Production via CO₂ Methanation
Methane synthesis, commonly referred to as methanation, is the reaction that combines CO₂ with hydrogen to yield methane and water:
CO₂+4H₂→CH₄+2H₂O\text{CO₂} + 4\text{H₂} \rightarrow \text{CH₄} + 2\text{H₂O}CO₂+4H₂→CH₄+2H₂O
Methanation is exothermic, meaning it releases energy, and is thermodynamically favorable. Catalysts like nickel are often used to increase reaction efficiency. This process produces synthetic methane, which can be injected into natural gas pipelines or used in gas-powered vehicles, further contributing to emissions reduction by displacing fossil fuel-derived methane.
Step 5: Renewable Hydrogen Production – The Backbone of Carbon Conversion
To convert CO₂ into synthetic fuels sustainably, an ample supply of green hydrogen is essential. Currently, around 96% of hydrogen is derived from fossil fuels, with a mere 4% generated via renewable energy-powered electrolysis, known as green hydrogen. Hydrogen produced via renewable electricity not only reduces the carbon footprint but also bolsters the environmental sustainability of CO₂ conversion processes.
Power-to-Gas and Power-to-Methanol Pathways
The production pathways are often named based on the synthetic fuel being produced. When renewable hydrogen is used to generate methane, the process is termed "power-to-gas," while the production of methanol from CO₂ and renewable hydrogen is referred to as "power-to-methanol." Each of these pathways offers a carbon-neutral or even carbon-negative solution, depending on the energy source for hydrogen production.
Advancements and Innovations in Hydrogen Production
In addition to green hydrogen, "blue hydrogen" has gained interest due to its potential to reduce CO₂ emissions. Blue hydrogen is derived from fossil fuels but involves capturing and storing the CO₂ emissions, reducing the overall carbon impact. Although blue hydrogen is not entirely carbon-neutral, it offers a lower-emission alternative, especially where renewable hydrogen production infrastructure is limited.
Environmental and Economic Implications
Reducing Fossil Fuel Dependency
Utilizing captured CO₂ to produce synthetic fuels not only offsets emissions but also reduces dependency on fossil-derived raw materials. Synthetic methane and methanol can substitute for natural gas and petroleum-based methanol, creating a more circular and sustainable energy economy.
Boosting Economic Resilience
This transition to low-carbon fuels strengthens economic resilience by reducing exposure to fossil fuel market volatility. Furthermore, developing CO₂-to-fuel technologies can create new job opportunities and support economic growth in sectors dedicated to renewable hydrogen and carbon capture.
Challenges and Future Outlook
Despite its promise, CO₂ conversion to synthetic fuels faces certain technical and economic challenges:
- High Costs: Green hydrogen production via electrolysis remains expensive, making CO₂ conversion economically challenging without subsidies or advances in electrolysis efficiency.
- Infrastructure Development: Large-scale deployment requires significant infrastructure investments, particularly in hydrogen production, compression, and CO₂ transport.
- Catalyst Efficiency and Durability: Developing more efficient and durable catalysts for methanol and methane production is essential to reduce costs and enhance long-term viability.
- Market Demand: The adoption of synthetic fuels depends on demand in sectors like transportation, which may require policy support for widespread acceptance.
However, with continued research, technological advancements, and policy incentives, CO₂ conversion to synthetic fuels could become more commercially viable, contributing to a cleaner and more sustainable energy future.
Conclusion
Harnessing green hydrogen to convert carbon emissions into valuable resources like methane and methanol offers a promising avenue for reducing CO₂ emissions and transitioning toward a circular carbon economy. Through innovative capture, transportation, and conversion processes, industries can utilize CO₂ as a resource, helping to mitigate climate change while producing low-carbon synthetic fuels. As technology and infrastructure continue to advance, this approach could play a pivotal role in achieving global decarbonization targets and securing a sustainable energy future.