Thermocyclic Ammonia Production: A Sustainable Path for Hydrogen Storage and Transport
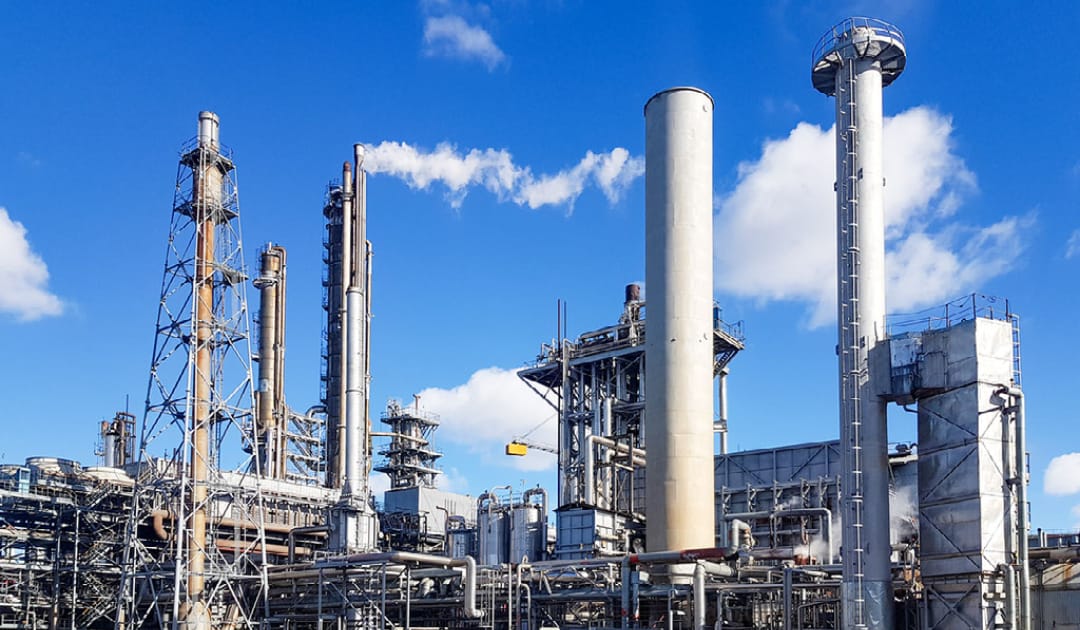
A Deep Dive into Thermocyclic Ammonia Production
Introduction
Thermocyclic ammonia production represents an innovative approach in energy storage and transport, positioning ammonia as an efficient hydrogen carrier. This process envisions a cyclical system where ammonia is synthesized, transported, and later decomposed back into nitrogen and hydrogen, enabling it to function as a hydrogen reservoir. This concept holds significant promise as a sustainable energy solution, especially in the face of mounting global efforts to reduce carbon emissions and establish reliable hydrogen supply chains.
Understanding Thermocyclic Ammonia
Thermocyclic ammonia production involves two primary stages:
- Ammonia Synthesis: This step traditionally uses the Haber-Bosch process, a well-established method that combines nitrogen (N₂) and hydrogen (H₂) under high pressure and temperature with an iron-based catalyst.
- Ammonia Decomposition: This process reverses synthesis by breaking down ammonia (NH₃) back into nitrogen and hydrogen. Since ammonia decomposition is endothermic, it requires a significant energy input, which underscores the importance of sustainable energy sources for the process to be eco-friendly.
The dual nature of thermocyclic ammonia production—synthesis followed by decomposition—allows ammonia to act as a vector for storing and transporting hydrogen. This capability offers advantages in energy applications where pure hydrogen's lower energy density and handling difficulties make direct transport less efficient.
Potential of Thermocyclic Ammonia in Energy Systems
Countries aiming to import hydrogen can consider thermocyclic ammonia as a practical medium for safe hydrogen transportation. By shipping ammonia and decomposing it on-site, hydrogen can be released without the complications of transporting it in its pure form. This potential has piqued the interest of nations pursuing low-carbon energy imports, as it aligns well with global sustainability goals and the need for efficient hydrogen logistics.
Key Considerations for a Thermocyclic Ammonia System
*. Efficiency of the Process
The success of thermocyclic ammonia hinges on the system's overall efficiency. Both synthesis and decomposition steps must be optimized to reduce energy losses, given that ammonia production (via the Haber-Bosch process) and decomposition are energy-intensive. Improvements to energy efficiency could drive the adoption of thermocyclic ammonia as a viable hydrogen storage option.
*. Catalyst Innovation
The Haber-Bosch process is facilitated by catalysts that promote ammonia synthesis at high temperatures and pressures. Ongoing research aims to develop catalysts capable of operating at milder conditions, which would reduce the energy demands of synthesis. The availability of low-temperature and pressure catalysts could be transformative, enhancing the efficiency and sustainability of thermocyclic ammonia systems.
*. Ammonia Decomposition Methods
A variety of techniques for ammonia decomposition exist, each with specific benefits and limitations. For instance:
- Thermal Decomposition: Heating ammonia to high temperatures facilitates decomposition but requires significant energy, which poses efficiency challenges.
- Catalytic Decomposition: Using catalysts to lower the decomposition temperature improves efficiency. Innovations in catalysts, including those made from platinum, ruthenium, and nickel, are central to increasing reaction rates and lowering energy demands.
- Plasma Decomposition: Plasma technologies can decompose ammonia under relatively mild conditions, but scalability remains a key issue.
The selection of decomposition technology impacts system cost, efficiency, and environmental footprint. Future advancements in catalytic and plasma-based methods are particularly promising in making ammonia decomposition more economical and sustainable.
*. Integration with Renewable Energy Sources
To ensure a low-carbon footprint, thermocyclic ammonia systems should rely on renewable energy sources. Solar, wind, and hydroelectric power offer sustainable options for generating the energy needed in synthesis and decomposition steps. However, renewable energy sources are often intermittent, raising the need for energy storage solutions that can stabilize power supply and maintain continuous ammonia production and decomposition cycles.
One potential solution is energy buffering through batteries or supercapacitors that store renewable energy when production is high and release it during lulls. Alternatively, advanced demand-side management techniques, like peak load shifting, could help synchronize ammonia production with renewable energy availability.
Advanced Considerations for Thermocyclic Ammonia Systems
*. Ammonia Decomposition Technologies and Efficiencies
The efficiency of ammonia decomposition is key to the viability of thermocyclic systems. Research is ongoing to develop more effective catalysts and decomposition techniques, especially those that work under milder temperatures and pressures. Catalysts such as nickel-based or ruthenium-supported options show promise for low-temperature decomposition, enhancing the overall thermodynamic efficiency of the process.
*. Life-Cycle Analysis of Thermocyclic Ammonia
Conducting a life-cycle analysis (LCA) can provide insights into the environmental and economic impacts of thermocyclic ammonia systems. An LCA would evaluate carbon emissions, energy consumption, and resource use across synthesis, transportation, decomposition, and hydrogen extraction phases. Understanding the cradle-to-grave environmental footprint is essential for gauging the sustainability of thermocyclic ammonia as a hydrogen carrier.
Economic considerations also play a role, as cost-intensive steps like ammonia decomposition may require financial incentives or subsidies to compete with traditional hydrogen transport methods. Policymakers can use LCA results to design support mechanisms for thermocyclic ammonia adoption.
*. Technological Challenges and Research Frontiers
- Scaling Catalyst Production: Efficient catalysts for low-temperature synthesis and decomposition remain in the research phase, and scaling these solutions for commercial applications is challenging.
- Efficient Ammonia Synthesis: Despite progress, the Haber-Bosch process remains energy-intensive. There is ongoing research into alternative processes, such as electrocatalytic ammonia synthesis, which might offer lower energy consumption under mild conditions.
- Ammonia Decomposition Advancements: Innovative decomposition methods, including microwave-assisted and photocatalytic processes, could make decomposition less energy-intensive, aligning with renewable energy use and reducing the overall process costs.
Environmental Implications of Thermocyclic Ammonia
Thermocyclic ammonia can contribute to a more sustainable energy system by lowering the carbon footprint of hydrogen transport. However, this requires that both synthesis and decomposition steps are powered by renewable sources. Moreover, ammonia itself is toxic, and safe handling practices are essential to prevent environmental harm.
Policy support and robust regulatory frameworks could address these environmental challenges, ensuring thermocyclic ammonia systems adhere to strict safety and sustainability standards.
Practical Applications of Thermocyclic Ammonia
*. Hydrogen Transport for Importing Nations
Many countries view hydrogen imports as a cornerstone of their energy strategy, particularly those with limited domestic renewable resources. By shipping ammonia and decomposing it into hydrogen at the destination, importing countries can leverage hydrogen without the storage and transport issues associated with cryogenic or compressed hydrogen.
*. Ammonia as a Renewable Fuel Source
Apart from its role as a hydrogen carrier, ammonia can serve as a direct fuel source, especially in sectors that are difficult to electrify, such as maritime shipping. The thermocyclic approach provides flexibility, allowing ammonia to be used either directly or as a hydrogen source, depending on demand.
Conclusion
Thermocyclic ammonia production represents a groundbreaking pathway for sustainable hydrogen transport and storage. By employing a cyclical approach where ammonia is synthesized, transported, and decomposed back into its constituent elements, thermocyclic ammonia offers a practical solution for hydrogen distribution.
However, achieving a scalable, sustainable thermocyclic ammonia system requires technological advances, particularly in catalyst development, decomposition efficiency, and renewable energy integration. Continued research and development, supported by policies encouraging sustainable practices, could position thermocyclic ammonia as a central pillar in the global transition to clean energy.
The thermocyclic approach, with its capacity to facilitate efficient hydrogen storage and transport, holds the potential to advance sustainable energy goals while meeting the growing demand for low-carbon fuel solutions worldwide.