Comparison of Hydrogen Carrier Technologies for Renewable Energy Transportation - ammonia (NH₃) vs liquid hydrogen (LH₂) vs Liquid Organic Hydrogen Carriers (LOHCs) vs methanol (CH₃OH)
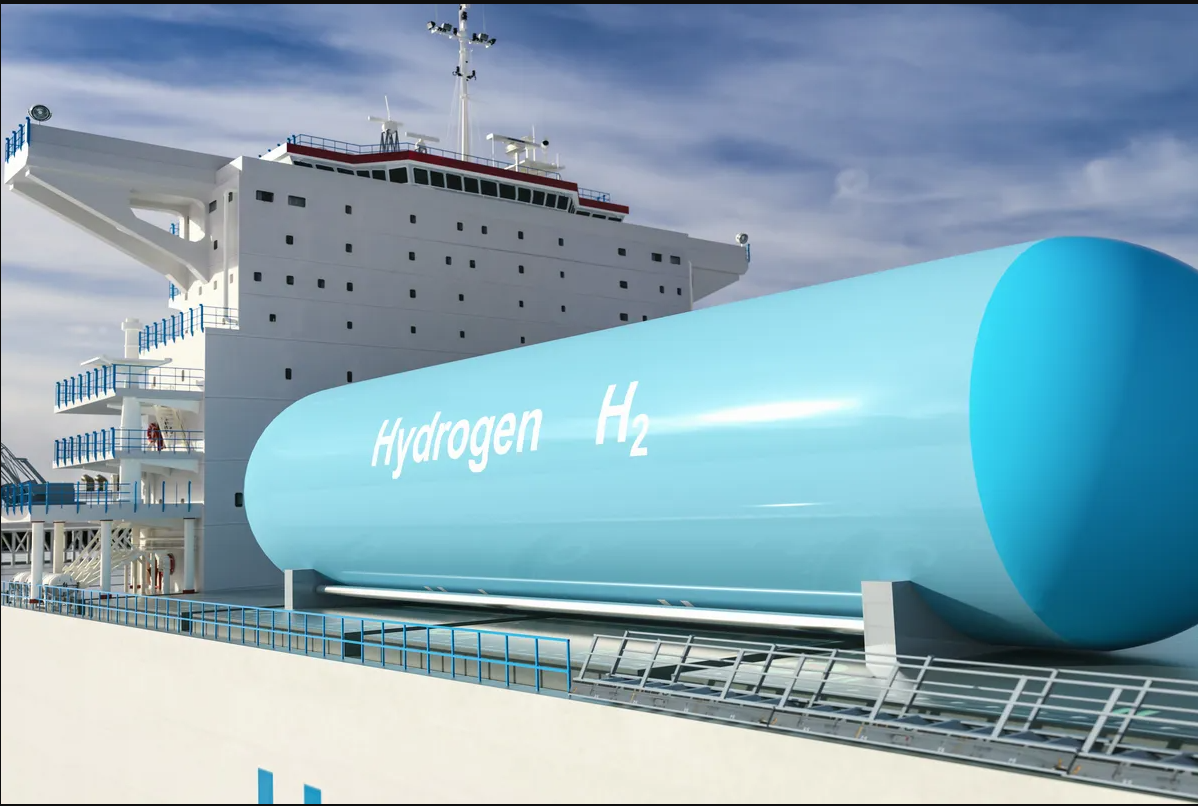
As the global transition to renewable energy accelerates, hydrogen is emerging as a critical player in the decarbonization of various industries. However, the effective transport of hydrogen remains a key challenge, particularly when moving it over long distances between regions or continents. Hydrogen carrier technologies provide solutions to this challenge by enabling the transport of hydrogen in various chemical forms, each with its own advantages and limitations.
This report explores the primary hydrogen carriers currently being considered for renewable energy transportation: ammonia (NH₃), liquid hydrogen (LH₂), Liquid Organic Hydrogen Carriers (LOHCs), and methanol (CH₃OH). Each of these carriers presents distinct characteristics in terms of production processes, infrastructure requirements, and technical considerations. The choice of carrier has a significant impact on the energy efficiency, cost, and sustainability of hydrogen transportation, making it crucial for stakeholders to evaluate these factors when determining the optimal carrier for specific scenarios.
1. Ammonia (NH₃)
Ammonia is one of the most promising hydrogen carriers for large-scale transportation due to its relatively high hydrogen content and the existing global infrastructure for its production, storage, and transport.
1.1 Production
Ammonia is synthesized using renewable hydrogen and nitrogen (extracted from the air) through the Haber-Bosch process, a well-established industrial process. In renewable ammonia production, hydrogen is produced via electrolysis using renewable energy sources like solar and wind, ensuring that the entire process is emissions-free. Nitrogen is readily available from the atmosphere, making ammonia production dependent mainly on the availability of renewable hydrogen.
1.2 Infrastructure
Ammonia benefits from an extensive existing global infrastructure, including storage tanks, pipelines, and shipping terminals. Many ports worldwide already have ammonia handling capabilities, which can be leveraged in the early stages of green ammonia production. As demand for green ammonia grows, additional investments will be required to expand transportation and storage capacity.
One key advantage of ammonia as a hydrogen carrier is its ability to utilize existing infrastructure designed for hydrocarbons, such as diesel. This reduces the risk of asset stranding, where infrastructure investments become obsolete due to changes in energy sources.
1.3 Technical Considerations
Ammonia has a high energy density and hydrogen content, making it efficient for transporting large quantities of hydrogen. It is also versatile, capable of serving as both a hydrogen carrier and a fuel for various applications, including power generation and marine transportation.
However, ammonia poses certain challenges, particularly in its toxicity and flammability. Its direct use as a fuel requires addressing safety concerns and the development of technology for ammonia combustion. Additionally, dehydrogenation—the process of extracting hydrogen from ammonia—requires energy-intensive cracking processes at the destination, which can reduce overall energy efficiency. Despite these challenges, the potential to repurpose existing infrastructure makes ammonia a strong candidate for hydrogen transportation, particularly for long-distance or intercontinental trade.
2. Liquid Hydrogen (LH₂)
Liquid hydrogen (LH₂) is another key technology for transporting hydrogen, particularly for applications that require high-purity hydrogen. However, the cryogenic nature of liquid hydrogen introduces specific challenges related to infrastructure and energy efficiency.
2.1 Production
Liquid hydrogen is produced by cooling gaseous hydrogen to -253°C, a process that requires significant energy input. The liquefaction process demands high-purity hydrogen, making it a resource-intensive method of hydrogen transportation. However, once liquefied, hydrogen can be stored and transported at much higher densities than in its gaseous form.
2.2 Infrastructure
Liquid hydrogen transportation faces considerable infrastructural constraints. The cryogenic nature of liquid hydrogen requires specialized facilities, including liquefaction plants, storage tanks, and ships equipped with advanced insulation systems. Currently, global infrastructure for liquid hydrogen transport is limited, and significant investments are needed to scale up the necessary infrastructure for long-distance transportation.
The cryogenic storage requirements for liquid hydrogen also mean that boil-off losses—where a portion of the hydrogen evaporates due to warming—must be carefully managed, further complicating storage and transportation. Nonetheless, advancements in cryogenic technology and insulation systems are helping to mitigate these challenges, making liquid hydrogen a feasible carrier for certain applications.
2.3 Technical Considerations
Liquid hydrogen boasts a higher density than compressed gaseous hydrogen, making it more efficient for transportation in terms of volume. However, the energy-intensive liquefaction process significantly reduces the overall energy efficiency of using liquid hydrogen as a carrier. Additionally, maintaining the cryogenic temperature over long distances presents technical and economic challenges, as specialized storage and transport systems are required to prevent boil-off.
Despite these challenges, liquid hydrogen offers distinct advantages in terms of its purity and the potential to supply high-demand industries like fuel cells and aerospace applications, which require high-purity hydrogen.
3. Liquid Organic Hydrogen Carriers (LOHCs)
Liquid Organic Hydrogen Carriers (LOHCs) offer a flexible and efficient solution for hydrogen transportation, utilizing organic compounds that can reversibly bind with hydrogen.
3.1 Production
LOHCs are organic compounds that can absorb and release hydrogen through reversible hydrogenation and dehydrogenation processes. In the hydrogenation process, hydrogen is loaded into the carrier at the production site, and at the destination, dehydrogenation releases the hydrogen for use. LOHCs themselves are not consumed in the process and can be reused, offering a potentially sustainable transportation method.
3.2 Infrastructure
One of the key advantages of LOHCs is their compatibility with existing oil infrastructure, including pipelines, tankers, and storage tanks. Because LOHCs exhibit similar properties to hydrocarbons, they can be transported using much of the infrastructure already in place for oil and gas, which reduces the need for large-scale new investments.
LOHCs also offer a practical solution for storing and transporting hydrogen without significant boil-off losses. Unlike liquid hydrogen, LOHCs do not require cryogenic storage conditions, making them more cost-effective and easier to handle during transport and storage.
3.3 Technical Considerations
LOHCs provide a stable and safe method for hydrogen transportation, as they are not flammable or explosive, reducing safety risks compared to liquid hydrogen or ammonia. However, the energy required for the dehydrogenation process at the destination can be significant, which may offset some of the energy efficiency benefits gained during transport. Nevertheless, the ability to use existing infrastructure and the low boil-off losses during transportation make LOHCs an attractive option for hydrogen transport, particularly over long distances.
4. Methanol (CH₃OH)
Methanol is a versatile chemical that can serve as both a hydrogen carrier and a fuel. Its existing global infrastructure and the potential for renewable methanol production make it an appealing option for hydrogen transportation.
4.1 Production
Renewable methanol can be produced through methanol synthesis using renewable hydrogen and captured CO₂. The CO₂ used in this process is typically sourced from industrial emissions or directly captured from the air, creating a carbon-neutral or even carbon-negative fuel, depending on the source of CO₂.
4.2 Infrastructure
Methanol benefits from a well-established global infrastructure, with over 100 major ports worldwide equipped to handle methanol. As methanol is a liquid at ambient temperature and pressure, it is easier to store and transport than hydrogen in its gaseous or liquid forms. This simplifies the logistics of hydrogen transportation and reduces costs associated with specialized storage and shipping infrastructure.
The extensive global infrastructure for methanol also supports its use as a chemical feedstock and fuel, further enhancing its appeal as a hydrogen carrier. In many cases, methanol can be directly used as a fuel for power generation or transportation, avoiding the need for dehydrogenation and thereby improving energy efficiency.
4.3 Technical Considerations
Methanol’s versatility as both a hydrogen carrier and a fuel gives it a unique advantage. In addition to its role as a chemical feedstock, methanol can be converted back into hydrogen at the destination or used directly in fuel cells and combustion engines.
However, the sustainability of methanol depends heavily on the source of CO₂ used in its production. Capturing CO₂ from renewable sources, such as biomass or direct air capture, is essential to ensure that renewable methanol is a truly sustainable hydrogen carrier. Additionally, the energy required for the methanol synthesis process and the conversion of methanol back into hydrogen at the destination must be considered when evaluating its overall efficiency as a hydrogen carrier.
5. Comparing Hydrogen Carriers
The choice of hydrogen carrier technology depends on a variety of factors, including the distance of transportation, existing infrastructure, and the intended end-use of the hydrogen. Each carrier has distinct advantages and challenges that influence its suitability for specific transportation routes and applications.
- Energy Efficiency: Liquid hydrogen requires the most energy input for the liquefaction process at the production site. In contrast, ammonia, LOHCs, and methanol require significant energy for dehydrogenation or cracking at the destination. However, the direct use of ammonia and methanol as fuels or chemical feedstocks can mitigate the energy losses associated with hydrogen extraction.
- Infrastructure Compatibility: LOHCs and methanol benefit from the ability to use existing oil and chemical infrastructure, reducing the need for large-scale new investments. Ammonia also has the advantage of an established global infrastructure, while liquid hydrogen requires specialized and costly cryogenic facilities.
- Safety and Handling: LOHCs offer the safest option for hydrogen transportation, with low flammability and explosion risks. Ammonia and liquid hydrogen require careful handling due to their toxicity and cryogenic properties, respectively, while methanol presents fewer safety challenges given its stability as a liquid at ambient conditions.
- End-Use Flexibility: Ammonia and methanol offer significant versatility as both hydrogen carriers and direct-use fuels, making them attractive for industries such as shipping, chemicals, and power generation. LOHCs and liquid hydrogen, on the other hand, primarily serve as hydrogen carriers and are less suitable for direct fuel applications